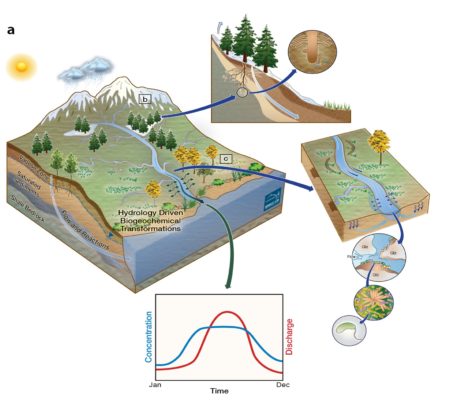
Figure: The Watershed Function project is developing and testing system-within-system, scale-adaptive approaches at the East River, CO Watershed to quantify how spatially variable hydrological-biogeochemical responses to perturbations propagate through the system and lead to an aggregated downgradient watershed discharge and concentration signature.
While watersheds are recognized as Earth’s key functional unit for managing water resources, their hydrological interactions also mediate biogeochemical processes that support all terrestrial life and can lead to a cascade of downgradient effects. The Watershed Function Scientific Focus Area is developing a scale-adaptive predictive understanding of how mountainous watersheds retain and release water, nutrients, carbon, and metals. This paper describes several recently developed approaches to interrogate, monitor and simulate transient watershed partitioning and biogeochemical responses – from genome to watershed spatial scales and from episodic to decadal timescales.
A growing demand for clean water, food, and energy — in parallel with droughts, floods, early snowmelt and other disturbances — are significantly reshaping interactions within watersheds throughout the world. This is particularly true for mountainous systems, such as the East River Watershed in CO, which is located in the Upper Colorado River Basin. This Basin supplies water to 1 in every 10 Americans and supports vast agriculture and hydropower operations along its reach. Because society is dependent on watersheds, new approaches that can accurately yet tractably predict watershed responses to disturbances are critical for resource management.
Summary
The Watershed Function project is developing new approaches to quantify and predict how disturbances impact downstream water availability and biogeochemical cycling, with a current focus on early snowmelt. The research is guided by a system-of-systems perspective and a scale-adaptive approach, where a predictive understanding of the response of archetypal watershed subsystems to disturbances is being developed as well as methods to aggregate such responses into predictions of cumulative watershed exports. The paper describes several recent advances, including above-and-below ground characterization and monitoring approaches for understanding vegetation distribution; new modeling approaches for predicting bedrock-through-canopy hillslope interactions; and coupled modeling approaches that can assimilate increasingly available streaming data into models to estimate hillslope water partitioning over time. The paper also describes new watershed function insights gained through the use of such tools, including: how historical snowmelt and monsoon characteristics influence annual discharge across the entire watershed; controls on streamflow generation; and how future changes in vegetation and temperature may influence water partitioning at different positions in the watershed. Over 30 institutions are involved in advancing watershed hydrological-biogeochemical science at the East River CO DOE-BER ‘community testbed’.
Citation
Hubbard, S.S. et al. (2018) The East River, CO Watershed: A Mountainous Community Testbed for Improving Predictive Understanding of Multi-Scale Hydrological-Biogeochemical Dynamics. Vadose Zone Journal, Special Issue on ‘Hydrological Observatories’, doi: 10.2136/vzj2018.03.0061 in press.